Definition, etymology and general principles
Mycotoxins were discovered more than 5,000 years ago in China, and in 1962 their effects became known for the first time after a devastating veterinary crisis near London, England, in which approximately 100.000 turkeys died (Blount, 1961). Subsequently, when this unknown illness was linked to peanut meal contaminated with secondary metabolites from Aspergillus flavus (aflatoxins), scientists at the time speculated that other mold metabolites could be lethal.
The classification of mycotoxins was soon extended to include a number of previously known fungal compounds (e.g. ergot alkaloids), for example, some compounds originally isolated as antibiotics (e.g. patulin) and a number of new secondary metabolites after screening of suspected molds harmful to human and animal health (e.g. ochratoxin A; Bennet et al., 2003).
It is difficult to define mycotoxins in a few words. All mycotoxins are low molecular weight natural products (i.e. small molecules) produced as secondary metabolites by filamentous fungi. These metabolites constitute a toxigenic and chemically heterogeneous assemblage, which can cause disease and death in humans and other vertebrates (Pitt et al., 2016).
The mycotoxins classification, as well as their definition, is extremely difficult due to the complexity of their chemical structure, their biosynthetic origin, their multiple biological effects and their production by a large number of fungal species.
However, to date, more than 400 mycotoxins have been identified, of which about a dozen groups are regularly highlighted as a risk to human and animal health (Agriopoulou et al., 2020).
Although the reasons for their production have not been revealed, it is thought that they are a defense mechanism against insects, as many mycotoxins and their metabolites have insecticidal properties (Richard, 2015).
Crops are particularly susceptible to contamination by mycotoxin-producing molds, and this may occur during harvesting (Diener et al., 1987), storage or transport (Smith et al., 1985).
Consequently, when animals feed on these contaminated products, they can eat the mycotoxins and these may produce toxic effects on them. Mycotoxin intoxications are known as mycotoxicoses. To prove that a disease is a mycotoxicosis, it is necessary to demonstrate a dose-response relationship between the mycotoxin and the disease and symptoms.
Mycotoxicosis symptoms depend on the type of mycotoxin; the amount and time of exposure; the age, health status and sex of the exposed individual.
Mycotoxins play a key role in animal performance, health and welfare; this has led to extensive research on this aspect including information on mycotoxin biosynthesis, mycotoxin producing species and clinical manifestations of each mycotoxin.
Characterisation and discovery of common mycotoxins
This group of toxins was discovered in the 1960s by veterinarian William Percy Blount (Figure 1), after the death of 100,000 turkeys in England. Based on a rigorous series of tests, ranging from the analysis of major bacterial toxins to a wide range of chemicals, pesticides and feed ingredients, Blount (1961) identified that the underlying cause of these tragic deaths was directly related to the birds’ feed.
Although the connection between the intoxication and the diet was confirmed, the accurate cause remained undefined.

Figure 1. William Percy Blount.
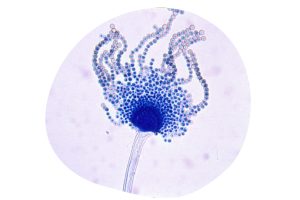
Figure 2. Aspergillus flavus.
At the same time, in Kenya, an alarming extended increase in cases of liver disease among chickens was observed, leading to the discovery of groundnut meal contaminated with Aspergillus flavus (Figure 2) from which it also takes its name.
J.J. Elphick, of the Commonwealth Mycological Institute in the United Kingdom, identified this mold due to the marked fluorescence of the AFs (Sargeant et al., 1961). At the beginning, the techniques used to study this fungus, included paper chromatography and later, thin layer chromatography was also used (Comes et al., 1963; Broadbent et al., 1963).
The decisive step forward in the identification of the origin of the AFs was made possible by the geographical proximity of the affected turkeys to the port of London. This allowed tracing of contaminated feed ingredients, in particular SS Rossetti flour which was shipped from Brazil in 7 July 1960.
In the following years, significant advances were made, such as the development of more reliable analytical methods and the identification of four AFs (B1, B2, G1 and G2) from their fluorescent colours in thin layer chromatography (Nesbitt et al., 1962; Pitt et al. 2016).
In the 1920s, mold-contaminated corn was associated with estrogenic symptoms in pigs in the United States (McNutt et al., 1928). The same feed was fed to both guinea pigs and rats, resulting in the development of uterine swelling in both species.
Christensen et al. (1965) succeeded in isolating the compounds present in moldy maize, thus identifying two structures: F-1 and F-2.
The identification of F-1 was termed ergosterol and could be confirmed by various chemical reactions. On the other hand, Urry et al. (1966) determined the chemical structure of F-2, later named ZEN due to its structure and the fungus that produces it (Fusarium graminearum; Figure 3).
A synthetic form of the metabolite α-ZAL, known as zeranol, was used as an anabolic agent in sheep, sows and other species in the USA (Diekman et al., 1989). However, in 1989, the European Union (EU) banned the use of zeranol.
Sows show a high sensitivity to this mycotoxin, mainly due to the effects that have been reported in the literature and which mainly affect the reproductive system (Figure 4).
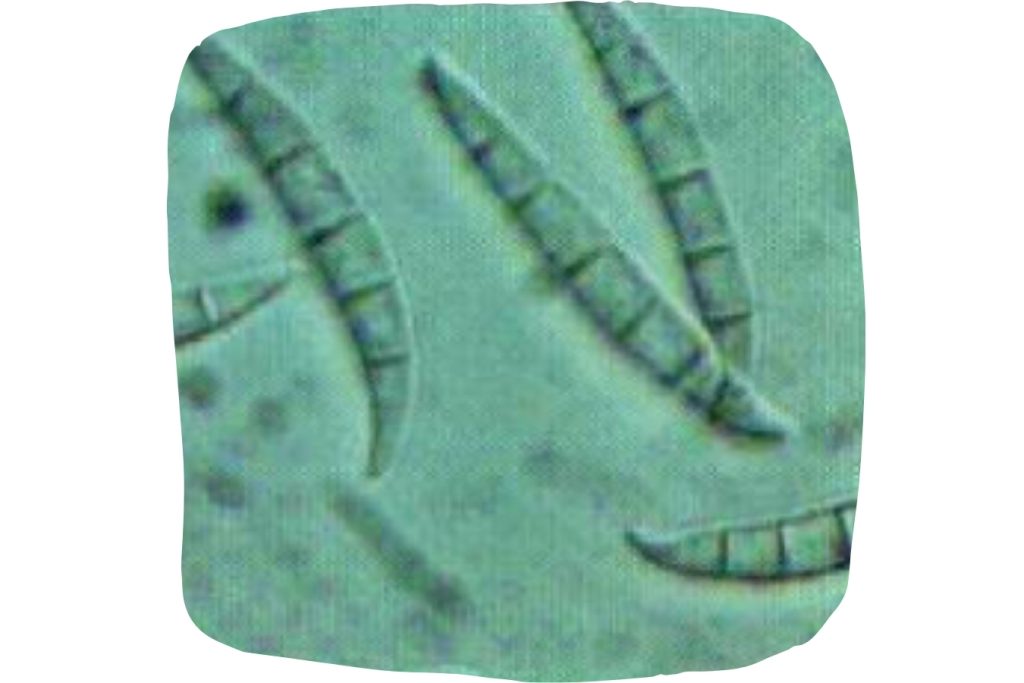
Figure 3. Fusarium graminearum.
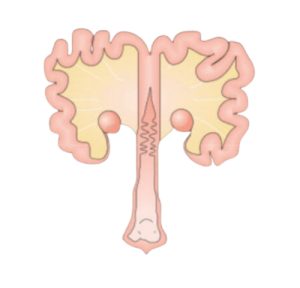
Figure 4. Sow reproductive system
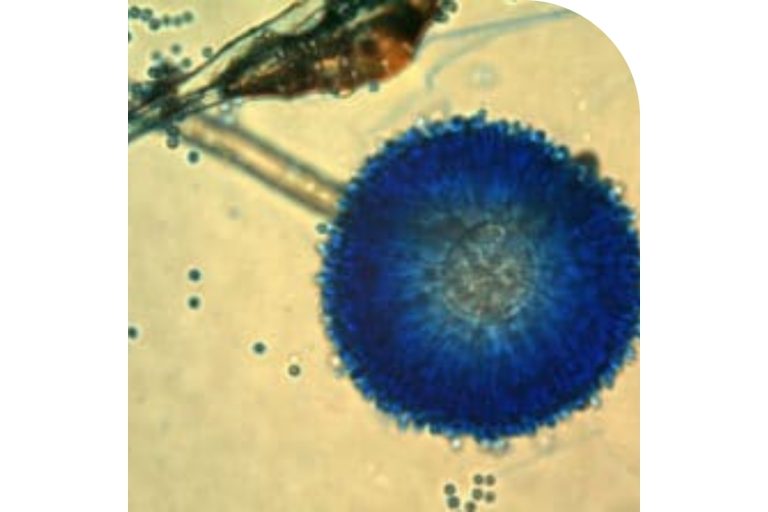
Figure 5. Aspergillus ochraceus.
OTA was isolated and characterized in 1965 in South Africa as a metabolite of the fungus Aspergillus ochraceus (Figure 5) during a large fungal metabolite study specifically designed to identify new mycotoxins (Van der Merwe et al., 1965).
This mycotoxin has effects mainly at the renal level, which has been described in all animal production species (Figure 6). In addition, effects on the liver, immune system and a potent immunosuppressive, teratogenic and carcinogenic effect have also been reported (Bennett et al., 2003).

Figure 7. Maize contaminated with Fusarium graminearum.
DON was discovered in 1970 in Japan (Yoshizawa et al., 1973), as a metabolite of Fusarium graminearum (Figure 7). Subsequently, Vesonder et al. (1973) published data on a trial in pigs and, due to outbreaks of emetic syndromes caused by DON in feed, this mycotoxin became commonly known as « vomitoxin, from vomiting ».
When ingested in high doses by farm animals, it causes nausea, vomiting and diarrhea; at lower doses, pigs and other farm animals show weight loss and food refusal (Rotter et al., 1996).
Canadian researchers have elucidated the toxigenic potential of F. graminearum, as well as the existence of two important chemotypes: 1) involved in DON production via 15-acetyldeoxynivalenol; and 2) via 3-acetyldeoxynivalenol (Greenhalgh, et al., 1984; Kasitu, et al., 1992).
T-2, produced by Fusarium sporotrichoides (Figure 8), was first isolated in 1930 in many cities of the former Soviet Union and is known to induce « Alimentary Toxic Aleukia » (ATA).
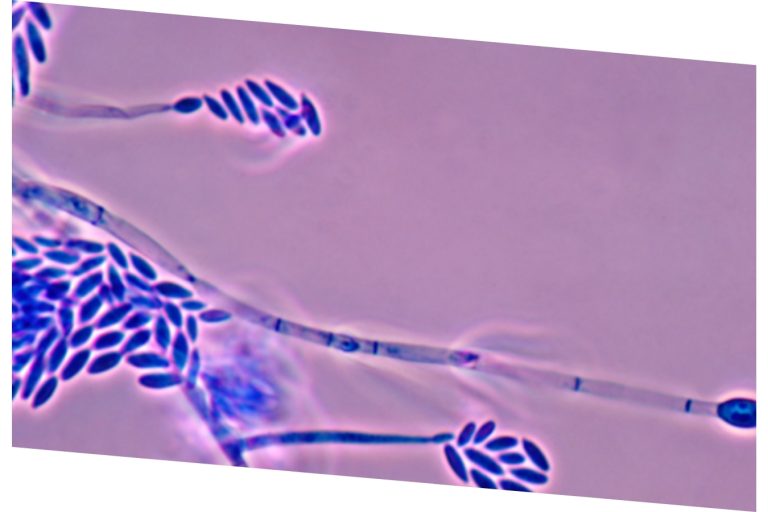
Figure 8. Fusarium sporotrichoides.
During World War II, due to the shortage of labor to cultivate the field, forage species were left in the field, contributing to increased exposure to food prepared with contaminated wheat. Subsequently, Russian scientists identified metabolites known as poaefusarin from Fusarium poae and sporofusarin from Fusarium sporotrichiodes (Joffe et al., 1971). Likewise, researchers at the University of Wisconsin succeeded in isolating T-2 in 1966 which was subsequently associated with the development of ATA (Mirocha et al., 1973).
FBs were discovered independently by two working groups in 1988.
One was investigating the cause of esophageal cancer in humans in South Africa (Bezuidenhout et al., 1988), whereas the other was working on the etiology of a well-known disease affecting horses, ELEM (leukoencephalomalacia) (Laurent et al., 1990). This group of toxins are produced by Fusarium spp. The best known are FB1 and FB2 and can affect animals in different ways by interfering with sphingolipid metabolism (Figure 9).

Figure 9. Structure of sphingolipids.
High concentrations of FB1 and FB2 can induce « swine pulmonary edema » (PPE) (Colvin et al., 1992; Prelusky et al., 1994). At low concentrations exposure to these toxins, hepatic and renal damage has been reported in piglets (Riley et al., 1993). In chickens, it has been described that the synergism of fumonisins together with other mycotoxins can induce the « lethal contamination syndrome » (Javed et al., 1993).
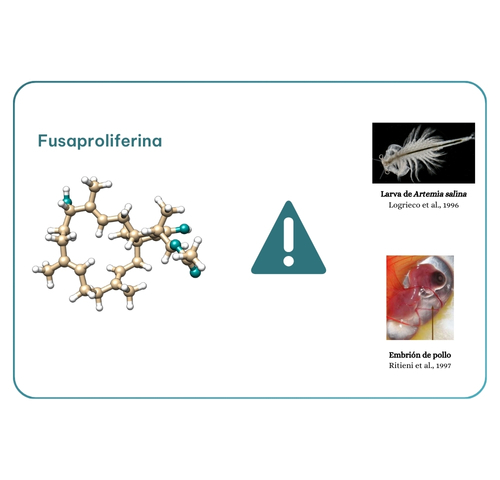
Figure 10. Fusaproliferin toxicity.
One of the first papers to use this term « emerging mycotoxins » was published in 2008 and focused on metabolites of Fusarium fusaproliferin (Jestoi et al., 2008). However, in a more recent paper, emerging mycotoxins were defined as « mycotoxins, which are neither routinely determined, nor legislatively regulated; however, evidence of their occurrence is rapidly increasing » (Vaclavikova et al., 2013). This group of mycotoxins includes fusaproliferin, beauvericin, enniatins and moniliformin, all produced by Fusarium spp.
Fusaproliferin (Figure 10), produced by F. subglutinans and F. verticillioides, was isolated in 1995 by researcher Ritieni. Toxicity data available are limited to Artemia salina larvae and chick embryos, including teratogenic effects (Logrieco et al., 1996; Ritieni et al., 1997).
Beauvericin (BEA; Figure 11) was isolated for the first time in 1991 in the United States (Gupta et al. 1991). Insecticidal, antimicrobial and antibiotic properties have been described.
Although in vitro studies have shown that BEA is toxic to rodents and poultry, these results have not been confirmed in vivo (Jestoi et al., 2008; Dornetshuber et al., 2009).
Enniatins (ENNs) were described by Gaumann and his research group in 1947 from the fungus Fusarium orthoceras.
Subsequent studies revealed a mixture of enniatins A (Figure 12), A1, B and B1 (Shemyakin et al., 1969) and a complex rich in enniatins A and A1 was identified as an active component in an insecticidal moiety targeting spruce budworm larvae (Strongman et al., 1988). Enniatins (ENNs) possess antibacterial, cytotoxic and oxidative characteristics, altering the microbial balance in the intestinal epithelium, potentially leading to secondary health effects (Dornetshuber et al. 2009).
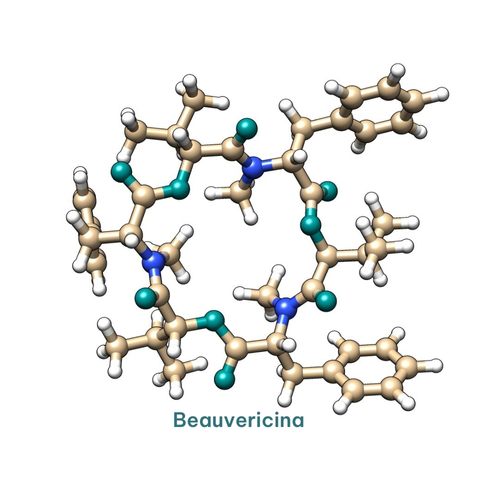
Figure 11. Beauvericina Structure.
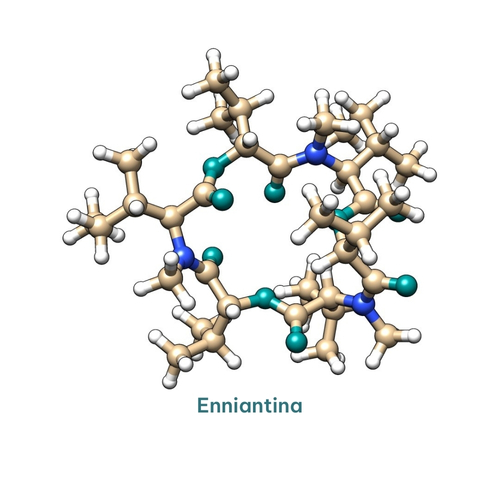
Figure 11. Enniantina Structure.
Mycotoxins derived from this group, are usually not detectable by conventional analytical techniques because their structure has changed are included in this group of mycotoxins.
For example, in studies designed to evaluate DON accumulation in corn, Miller et al. (1983) observed that DON concentration in field corn inoculated with F. graminearum decreased during the growing season.
The authors speculated that this decrease might be due to a chemical transformation of the toxin by plant enzymes. Shortly thereafter, the DON content of yeast-fermented food products was reported to be higher than that of contaminated wheat flour used for their production (Young et al., 1984). This increase was attributed to the enzymatic conversion of a DON conjugate generated by the metabolization of the toxin by wheat (Figure 13).

Figura 13. Biotransformación del DON
Furthermore, ZEN can be modified by microorganisms and plants, and these masked compounds can be completely converted into ZEN by animal or human intestinal microbiota. In humans and animals, the metabolism of ZEN can be divided into two phases, which include phase I metabolism and phase II metabolism (Zeng et al., 2019). ZEA-14-sulfate, is one of the compounds found in cultures of Fusarium graminearum in 1991, (Gareis et al., 1990) and results metabolism of ZEN (Figure 14).
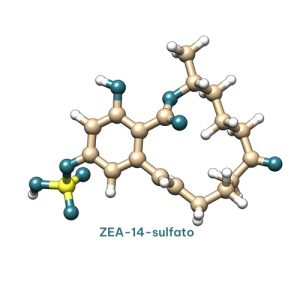
Figure 14. ZEA-14-sulfate structure.
Conclusions
Although the effects induced by mycotoxins have been studied for many years, research continues on their mechanism of action and on the strategies needed to mitigate their effects on human and animal health, agricultural losses and the potential effects of climate change.