Introduction
Fungi of the genus Fusarium are significant pathogens in agriculture and livestock, capable of causing various diseases in plants and animals. Among the most prominent diseases is Fusarium head blight, which primarily affects cereal crops. These diseases not only reduce crop quality and yield, but also have serious implications for animal and human health due to mycotoxin production. Over 200 Fusarium species have been classified, each with different pathogenic capabilities and mycotoxin production profiles. Among the most common species are Fusarium graminearum, Fusarium verticillioides, and Fusarium culmorum.
Fusarium Head Blight (FHB)
Fusarium head blight (FHB) is a disease caused by several species of Fusarium, particularly Fusarium graminearum. This disease affects cereals during the flowering phase, especially wheat and barley crops. Characteristic symptoms in the spikes include premature discoloration of infected spikelets, which can take over the entire spike causing significant economic losses. (Image 1)
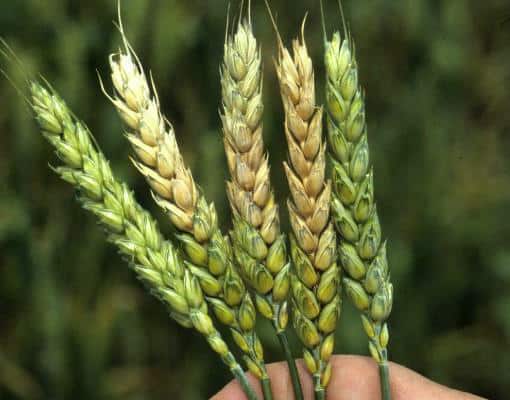
Image 1. Wheat infected with fusarium head blight
The propagation of Fusarium primarily occurs through spores transported by air or water, which infect the spikes of plants. The propagation methodology involves the germination of spores and penetration into plant tissues.
This expansion is favored in conditions of high humidity at the time of crop flowering and later during grain filling, which favor infection and disease development at warm temperatures between 22 and 28°C.
Fusarium expansion occurs through plant cell plasmodesmata. These are channels in the cell walls that connect the different plant cells. Image 2 shows how Fusarium crosses the cell walls through the plasmodesmata.
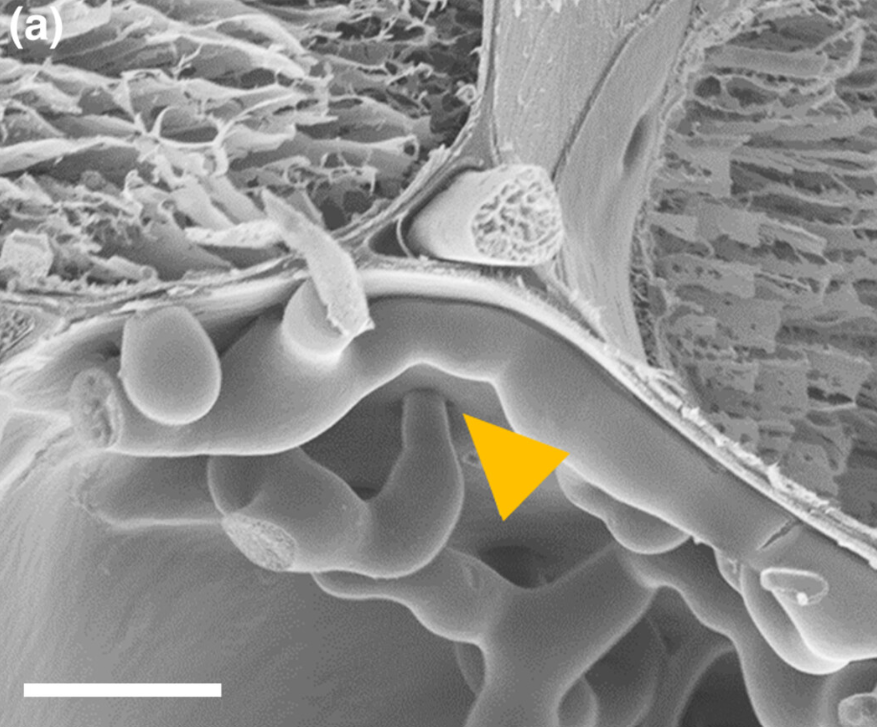
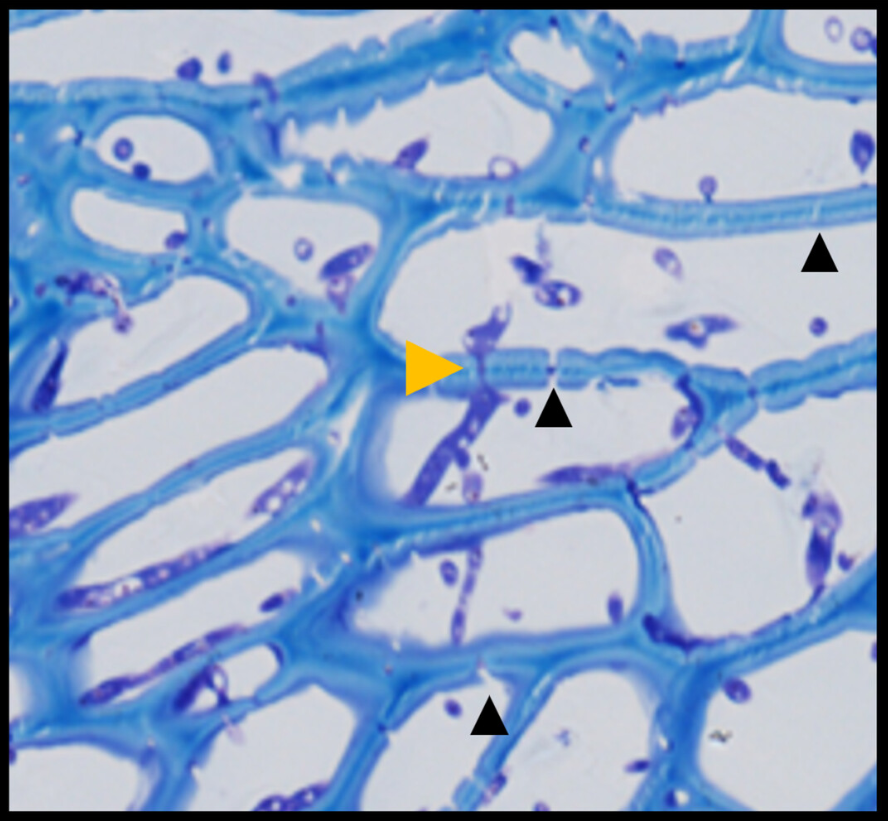
Image 2. a) Electron microscope photograph showing the Fusarium expansion in the plasmodesmata (yellow triangle)
b) Microscope photograph showing Fusarium crossing the cell wall through plasmodesmata (Lilac)
During the Fusarium expansion through plasmodesmata, cells activate a series of defenses to prevent or hinder expansion. These defense strategies include increasing the secretion of defense proteins and callose synthesis to block plasmodesmata, preventing spread to adjacent cells.
Therefore, to avoid defense mechanisms, fusarium synthesizes secondary metabolites, among them Deoxynivalenol (DON), which can inhibit the plant’s defenses, decreasing the cell’s protein synthesis and callosal deposition. This defense inhibition effect accelerates the expansion of the fusarium since plasmodesmata are not blocked by the deposition of callose (β-1,3-glucans). (Amer, Victoria. et al., 2024).
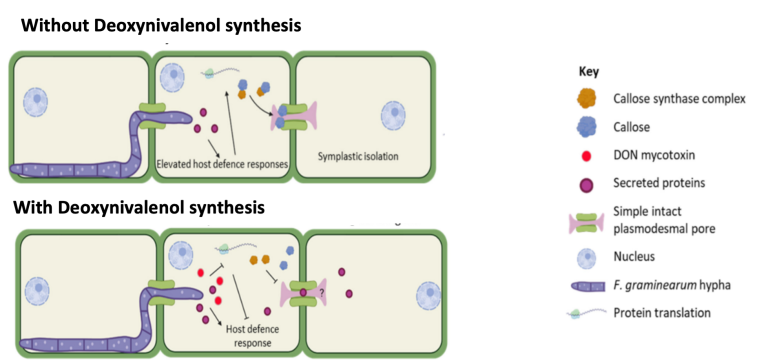
Image 3. Schematic of fusarium expansion without DON synthesis and with DON synthesis
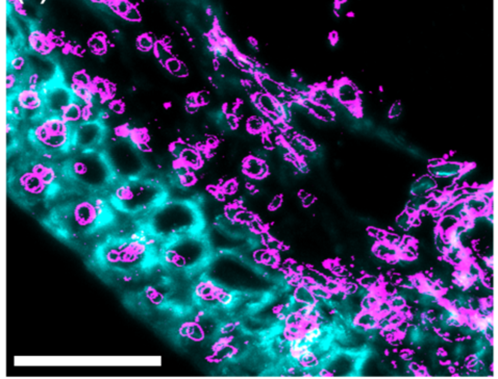
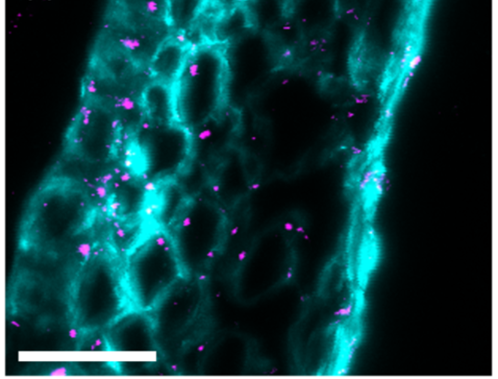
Image 4: Immunofluorescence photographs showing callosal deposition (pink) when the plant is facing fusarium attack: a) callose: deposition 5 days after fusarium infection, without trichothecene synthesis, b) callose: deposition, 5 days after fusarium infection, with trichothecene synthesis.
Mycotoxins generated by Fusarium
In addition to trichothecene, Fusarium can produce other mycotoxins, each with different effects on crops and animal health. Among the most important are:
Trichothecenes (Tri-A, Tri-B)
Deoxynivalenol (DON)
Effects on the plant:
- Growth inhibition: DON inhibits protein synthesis, which reduces root and shoot growth in plants.
- Cell damage: Causes the loss of chlorophyll and other photosynthetic pigments, leading to leaf bleaching and, eventually, necrosis.
- Loss of membrane integrity: DON increases plasma membrane permeability, causing electrolyte loss and cell death.
- Induction of apoptosis: It can trigger programmed cell death in plant cells through the accumulation of reactive oxygen species (ROS).
T2 and HT-2 Toxins
Effects on the plant:
- Inhibition of protein synthesis: These toxins interfere with ribosomal function, blocking protein synthesis and thus inhibiting plant growth.
- Morphological distortion: In plants such as Arabidopsis, T2 can cause dwarfism, twisted leaves, and other morphological deformities.
- Induction of oxidative stress: These toxins increase ROS levels, resulting in oxidative damage and cell death.
Fumonisins:
Effects on the plant:
- Interference in sphingolipid biosynthesis: Fumonisins inhibit ceramide synthase, a key step in sphingolipid synthesis, which alters cell membranes and cell signaling.
- Necrosis and chlorosis: The accumulation of toxic sphingolipid intermediates causes tissue necrosis and chlorosis in leaves, especially in corn.
- Reduced growth: Fumonisins have been observed to inhibit root elongation and seedling development.
Zearalenone (ZEN)
Effects on the plant:
- Alteration in membrane permeability: ZEN can modify plasma membrane permeability, causing electrolyte leakage.
- Inhibition of root growth: ZEN reduces ATPase activity, which negatively affects root growth in crops such as corn.
- Cellular acidification: Interferes with proton extrusion, leading to cellular acidification and affecting the plant’s overall metabolism.
Fusarin C:
Effects on the plant:
- Mutagenicity: Some types of fusarins, such as fusarin C, are mutagenic and can alter cell division in plants.
- Membrane damage: Fusarins can interfere with the integrity of cell membranes, leading to cell lysis and tissue death.
Moniliformin (MON):.
Effects on the plant:
- Inhibition of leaf development: Moniliformin reduces the effectiveness of photosynthetic pigments, which affects leaf development.
- Reduced biomass: It can reduce seedling mass and affect overall plant development.
- Moderate toxicity: Although it is less toxic than other mycotoxins, it still has a negative impact on plant growth.
Enniatins and Beauvericins (ENN and BEA):
Effects on the plant:
- Mitochondrial damage: These mycotoxins can induce apoptosis in plant cells by altering mitochondrial function.
Each of these mycotoxins has a specific toxicity profile. However, it is important to note that not all fusarium species are capable of producing all mycotoxins because their metabolic pathway is inhibited by enzyme deficiencies or mutations in metabolic pathways (Table 1).
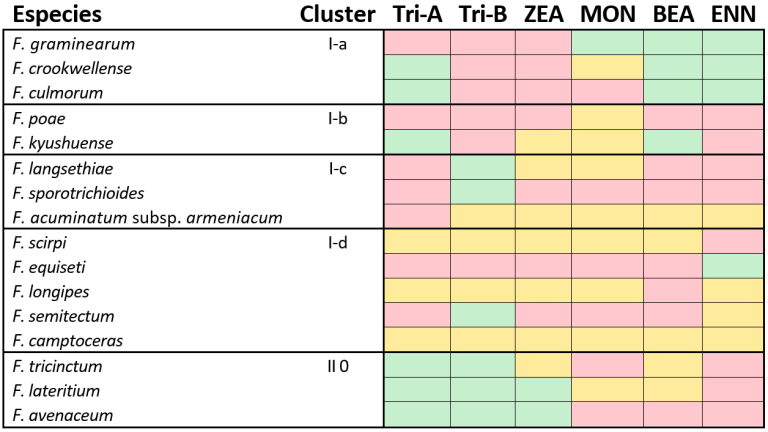
Table 1. Classification of Fusarium species that have the potential to produce mycotoxins. red= This species has the potential to produce mycotoxins, green= This species does not have the potential to produce mycotoxins, orange= This species may or may not produce mycotoxins, considered as missing data
The phylogenetic classification of the different species has allowed the different species to be grouped into 5 clusters (Subclass I-a, Subclass I-b, Subclass I-c, Subclass I-d and Subclass II) (image 5). It can be observed that there is a correlation between the different groups and the capacity of mycotoxin production. For example, that groups included in subclass II, subclass I-c and some species of subclass I-d cannot synthesize DON. (Watanabe M. et al., 2013)
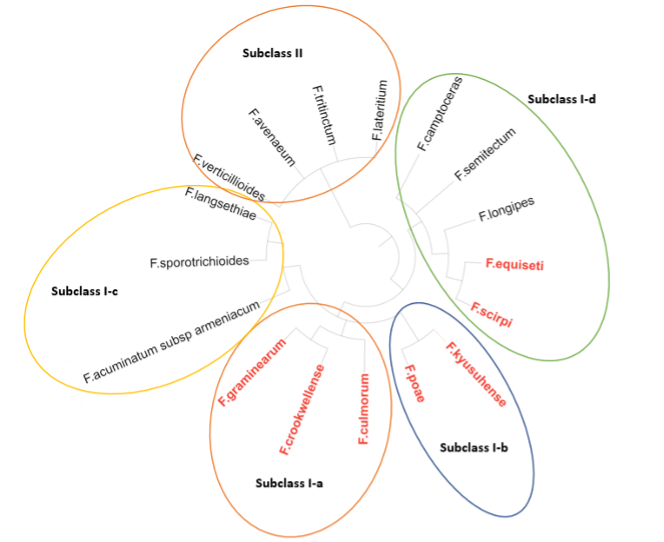
Image 5. Image of the phylogenetic classification of the different Fusarium species. It shows in red the producers of type B trichothecene (DON).
Metabolic pathways and trichothecene synthesis
Trichothecenes, including type A (T2 toxin, HT-2 Toxin) and type B (DON and Nivalenol), are synthesized through a single biosynthetic pathway shared in Fusarium. This metabolic pathway is regulated by a set of genes known as the «Tris Cluster» (Figure 6). Key genes in this cluster include Tri6 and Tri10, which are the regulators, and Tri13 and Tri7, which determine the trichothecene profile produced by the Fusarium strain. The variability in the synthesis of these trichothecenes may depend on environmental factors such as temperature and humidity, as well as the Fusarium species.

Figure 6 Group of TRIS trichothecene biosynthetic genes in Fusarium graminearum
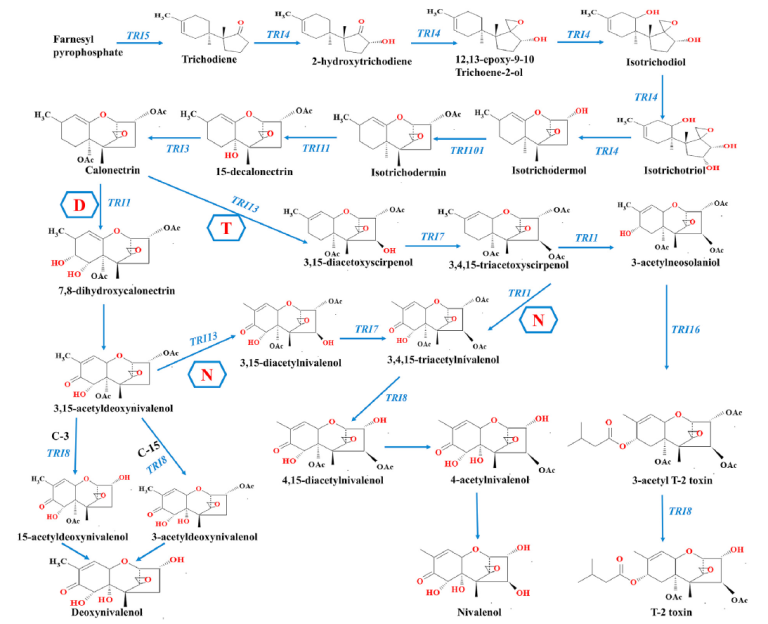
Image 7. Proposed pathways for trichothecene biosynthesis; N is the pathway in nivalenol-producing strains; D is the pathway in deoxynivalenol-producing strains; T is the pathway in T-2 toxin-producing strains. The trichothecene pathway was obtained from the MetaCyc pathway database
It is observed that the metabolic pathway is similar until the synthesis of calonectrin in which it will differentiate into TRI1 DON or TRI13 T2.
Nivalenol can be formed from the DON pathway by TRI13 in 3,15-acetyldeoxynivalenol or from the T2 pathway by TR11 in 3,4,15-tricocetoxyscirpenol. (image 7) and the production of this mycotoxin has been associated with greater stress factors.
The production of the type of trichothecenes synthesized can vary significantly depending on the species of Fusarium species and various factors such as regions, temperatures, agricultural practices… Studies have shown that Fusarium strains can modify their production of trichothecenes in response to specific environmental conditions, highlighting the importance of understanding the regulation of these genes to manage mycotoxin contamination. (Kolawole, Oluwatobi.et al., 2021) (Brown DW. et al., 2015)
Conclusions
In summary, the production of mycotoxins by Fusarium is a phenomenon that depends on both the species of the fungus and the environmental conditions. Some Fusarium species may not produce certain mycotoxins, while others may be highly productive under specific conditions. Understanding these factors is essential to developing effective prevention and control strategies in agriculture and animal nutrition.